Understanding the biologial journey of genetic inheritance can help in the interpretation of DNA evidence for genealogy research.
*This article originally appeared in NGS Magazine, and is reprinted with permission.
Different DNA inheritance paths can help solve genealogical mysteries. These unique inheritance patterns provide important context for interpretation of DNA test results and enable genealogical discoveries, but why is DNA inherited the way it is? What underlying biological processes explain the inheritance patterns of different types of DNA? What are the natural laws that govern genetic inheritance paths?
Genealogists commonly utilize historical, social, governmental and legal context to understand and interpret the records created regarding ancestors. Just as an understanding of the laws of a particular time period and place can aid in interpretation of records created for an ancestor, understanding the biological laws governing genetic inheritance can help in later interpretation of DNA evidence for genealogical purposes.
An Introduction to DNA

DNA provides the blueprint for all life and is written with four biological “characters” called bases: Adenine (A), Thymine (T), Guanine (G) and Cytosine (C). These bases are linked together in long strands to form an “instruction manual” for the human body. To protect this important biological code from damage, the strands of DNA align with complementary strands: A pairing with T and G pairing with C. The result is a double helix structure composed of millions of “basepairs.” A human genome (or a complete set of human DNA) contains approximately 6.4 billion basepairs.
Genetic Records, Archives, Transcription and Translation
The human genome is like an instruction manual that is divided into twenty-three “volumes” known as chromosomes. There are two “editions” of each volume of the instruction manual: a paternal edition inherited from an individual’s father, and a maternal edition inherited from an individual’s mother. The instruction manual also includes an “addendum” of mitochondrial DNA. The twenty-three chromosome volumes which form the majority of the genome instruction manual are housed as a single collection in an “archive” called the nucleus. The mitochondrial DNA addendum, meanwhile, is stored outside of the nucleus in locations called mitochondria.
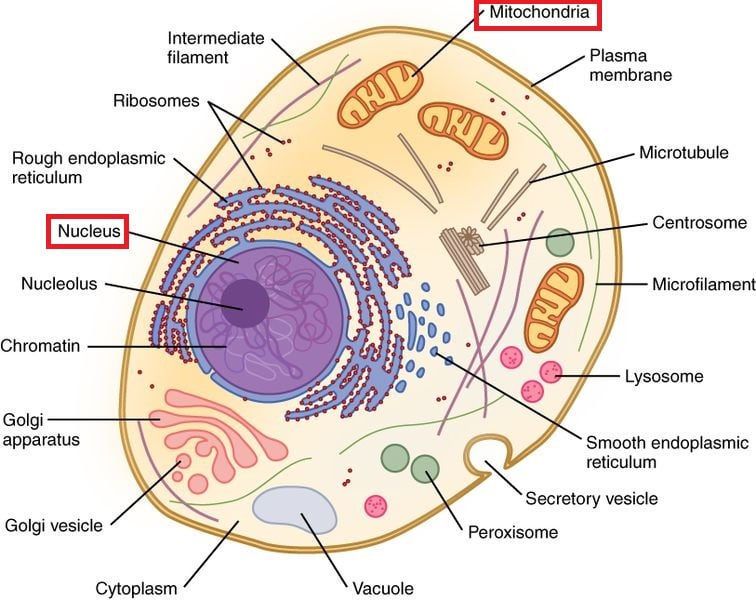
The human body is composed of trillions of cells and each of those cells (with a few exceptions) has a nucleus with a copy of the paternal and maternal editions of all twenty-three chromosome volumes. Each cell also has multiple mitochondria which serve as cellular powerhouses; each mitochondrion carries multiple copies of the mitochondrial DNA addendum. Though each cell contains a complete genome instruction manual, only parts of the manual are read depending on the type of cell. As with historic archives, consultation of the original DNA records is not possible outside of the nucleus archive. Instead portions of the DNA instructions read by specialized protein machines which “transcribe” select portions of the genome into RNA copies. These RNA transcriptions can be distributed outside of the archive and “translated” into sequences of amino acids. Chains of amino acids interact with each other to form proteins. The shape of proteins governs their function. Processes for reading, transcribing and translating mitochondrial DNA are similar to those employed in the nucleus.

Mitosis, Meiosis, Mutations
Human bodies grow, change, and develop through the continual replacement and division of cells – a process called mitosis. When a cell divides, the entire genome is copied. Each edition of each chromosome volume is replicated and bound with its duplicate at a part of the DNA strand called the centromere. Then, the nucleus is demolished. Next, a copy of each edition of each chromosome volume is distributed to opposite sides of the cell. Two new nuclei (archives) are built around these new copies of the instruction manual, and the cell then divides into two new cells. Thus, each daughter cell carries a complete copy of the genome. This process of genetic replication and division occurs millions of times each day.

Mitochondria replicate independently within each cell and contain multiple copies of mitochondrial DNA. When a mitochondrion becomes too large, it divides in half. Some copies of the mtDNA are included in one new mitochondrion and some copies are preserved in the other. When a cell divides, some mitochondria remain in one cell and other mitochondria remain in the other.
Given how often the genome is replicated, it is no surprise that sometimes errors are made in the replication of DNA. Some errors or mutations can have a detrimental effect and might lead to cell death. Others might affect the shape of a protein and its resulting function or prevalence. Other mutations have little to no effect and are passed on to descendant cells. The introduction of occasional mutations makes genetic genealogy possible. Any human individual shares approximately 99.9% of their DNA with all other humans. It is the .1% variation between humans and the associated inherited mutations that enable investigation, analysis and determination of the closeness of genealogical relationships.
Usually, when cells divide, a complete copy of the genome is passed on to both daughter cells. However, this is not the case for germ-line cells: sperm cells and egg cells. A process called meiosis results in sperm and egg cells with just a single edition of the genome rather than the two editions held by most other cells. Meiosis starts in much the same way as mitosis. Each chromosome is duplicated so that there are two copies of each edition of each of the twenty-three chromosome volumes. The two copies of each edition are bound together at the centromere. However, instead of separating to opposite sides of the cell, the paternal editions of each chromosome pair off with the maternal editions of each chromosome and become closely associated. A process called recombination often results in parts of a maternal edition of any given chromosome being swapped with corresponding parts of a paternal edition of the same chromosome.
After this exchange of information, the nucleus disintegrates, and the altered editions of each chromosome are pulled to opposite sides of the cell. One set of altered editions is pulled by its centromere to one side of the cell and the other set of altered editions is pulled by its centromere to the other side of the cell. The cell divides and the resulting cells divide again. During this second round of cell division, there is no replication of the editions and instead, an edition of each chromosome volume is pulled to opposite sides of the cell. The result of meiosis is four cells with a single edition of each chromosome volume – half the normal amount carried by most cells. In females, one large egg is created with three smaller cells which typically die. In males, four sperm cells are created. In any given cell the single edition of any given chromosome volume could be the maternal edition, could be the paternal edition, or could be (and often is) a compilation of information pulled from both the maternal and paternal edition of the chromosome.

Sex Chromosomes – Special Editions

Chromosome volumes 1-22 are very similar regardless of the edition consulted (maternal or paternal). The paternal and maternal editions of chromosome volume 23, meanwhile, can be quite different. In females, the paternal and maternal editions are X-chromosomes which are fairly similar. In males, meanwhile, chromosome volume 23 has two very different editions: A maternal X-chromosome edition and a much smaller paternal Y-chromosome edition. When meiosis occurs in females, the X-chromosomes line up and undergo normal recombination. The resulting four cells from the process each contain an X-chromosome. In males, meanwhile, the Y-chromosome and the X-chromosome line up but only undergo very limited recombination. Therefore, the Y-DNA and X-DNA are passed on essentially unchanged to daughter cells. Two of the four cells resulting from meiosis in males will contain Y-chromosomes and two will contain X-chromosomes.
Fertilization
During the process of human reproduction, millions of sperm cells approach an egg in a race to fertilize it. When an egg and a sperm combine, they form a zygote which has the potential to eventually develop into a human being – the next generation. If the sperm cell carried a Y-chromosome, then the resulting zygote could develop into a biological male. If the sperm cell carried an X-chromosome, then the resulting zygote could develop into a biological female.
While sperm cells do carry mitochondria and associated DNA, when the sperm cell penetrates the egg, the cellular contents of the sperm (including the mitochondria) are destroyed. Thus, except in rare cases, the only surviving mitochondrial DNA originates from the egg and is thus maternally inherited. The nucleus from the sperm, however, remains intact until it merges with the egg’s nucleus at which point the process of cell division can begin in the development of a fetus.
Genetic Inheritance and DNA: Y-DNA and X-DNA Inheritance
Y-DNA and X-DNA inheritance can be explained by the process of meiosis. In males, this process results in the creation of four sperm, two of which carry a Y-chromosome and two of which carry an X-chromosome. In females, the process of meiosis results in the formation of an egg cell which contains an X-chromosome. If the fertilizing sperm contains a Y-chromosome the resulting fetus will be male. If it contains an X-chromosome the resulting fetus will be female.
X-DNA inheritance and autosomal DNA inheritance can also be partially explained by the process of recombination in which each maternally inherited chromosome aligns with each paternally inherited chromosome and might exchange or shuffle genetic material into novel chromosome variants or editions. As a result, while each individual inherits 50% of their DNA from each parent in the form of a complete set of paternal and maternal chromosomes. Inheritance from more distant generations of ancestors is more random. For this same reason, X-DNA might, but may not necessarily be inherited from a specific subset of ancestors related through maternal lines or alternating maternal-paternal lines.
Mitochondrial DNA inheritance can be explained by the mechanics of fertilization which preserve mitochondria and associated DNA from egg cells but destroy mitochondria and associated DNA from paternal sperm cells.
Understanding these basic laws of genetic inheritance can help in the interpretation of DNA evidence for genealogy research.
Do you have a genetic genealogy mystery you would like help resolving? Contact Legacy Tree Genealogists today. Our team is experienced at utilizing DNA evidence from all major testing companies in combination with thorough records research to break down the genealogy “brick walls” in your family tree.
Hello Paul
Is a viable polyspermy in humans possible? That is, that a healthy baby is born whose genetic make up comes from the mother and from two male sperms? If it is, how rare is this. And is it possible to further ascertain this other than just having the genetic results revealing ethnic make up from both dads? Or is this completely impossible and absurd and the coincidence (of having ethnic make up from two possible dads) explained in some other way. Sorry if this is a stupid question!
Hi Judita,
Thank you for your questions, although they are a bit too complex to discuss in a blog reply. Please consider scheduling a consultation with our Client Solutions Specialists on our Get in Touch page. They can point you in the right direction or set up a research project if needed.